Carbon Capture and Catalytic Unitizations
Today, the atmospheric carbon dioxide (CO2) concentrations have reached an unprecedented record high of almost 420 ppm since the industrial revolution, resulting in an increase of the average global temperature by 1.5 ◦C. Tremendous efforts are being made to reduce carbon emission e.g. at stationary point sources such as fossil fuel-based power plants via CO2 capture and utilization (CCU) technologies. Here, we devote ourselves to the innovations of dual-functional materials that are able to capture CO2 and convert it to value-added chemicals in situ, including methane, methanol, carbon monoxide, etc. Such integrated systems eliminate the most energy & capital demanding upstream operations, such as stripping, compression, and transportation.
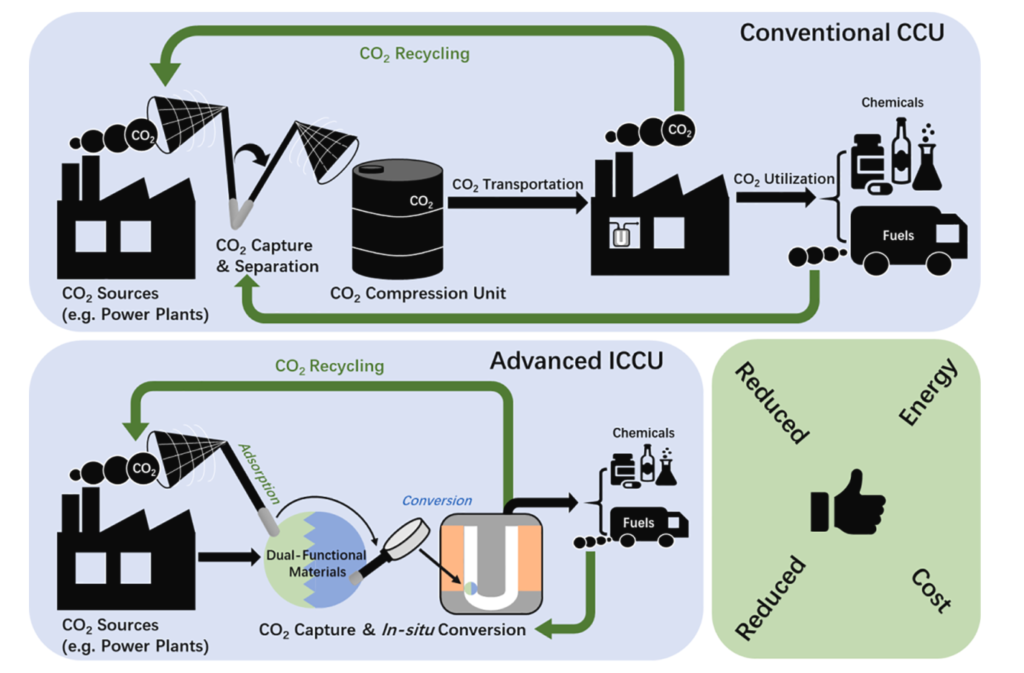
Structure-Property Relationship Studies
When the particle size shrinks to nano domain, most physiochemical characteristics including catalytic properties will become structure-sensitive, as the distribution of density of states in the electronic structure of the materials can be size/shape/facet dependent. The electronic structure of a catalyst is of vital importance to its catalytic properties. For example, in photocatalysis, it directly determines the absorption of photons that can be harvested to drive the reaction. In heterogenous catalysis, it affects the molecular adsorption process on the catalysts and consequently the reactivity. Therefore, understanding how the structure of a catalyst affects the catalytic property is central to achieve property engineering in the optimization of catalytic performance.
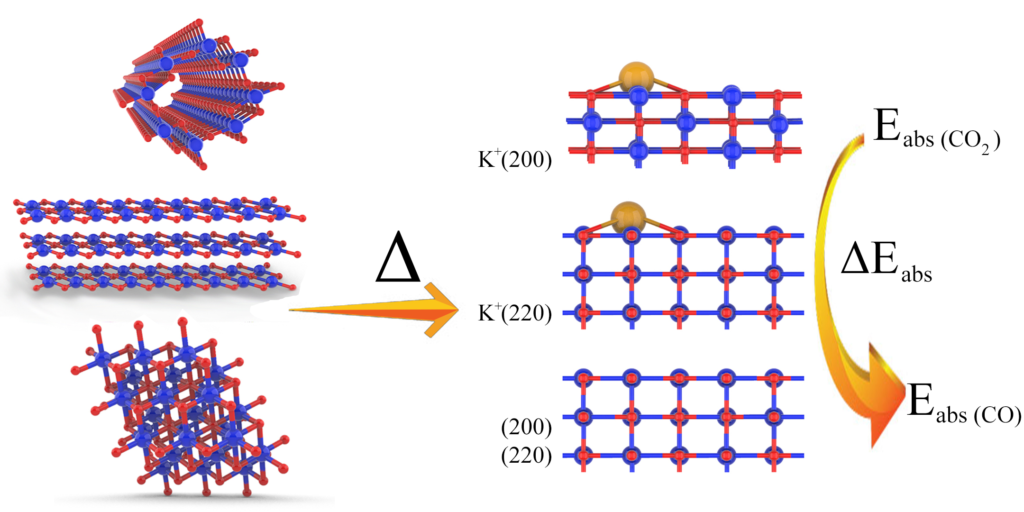
Controlled syntheses of catalyst nanostructures
The studies of fundamental structure-property relationships should only be conducted with well-defined catalytic systems to avoid multifactorial interference. Different solution-based wet-chemical processes will be implemented for the syntheses of metal and metal oxide nanostructures with finely controlled sizes, compositions, shapes, and facets, including surfactant-assisted soft-templating strategy, hard-templating process, colloidal syntheses, etc. We design catalysts, precious or nonprecious components, single or hybrid, organic or inorganic, with inspirations from natural enzymatic structures.
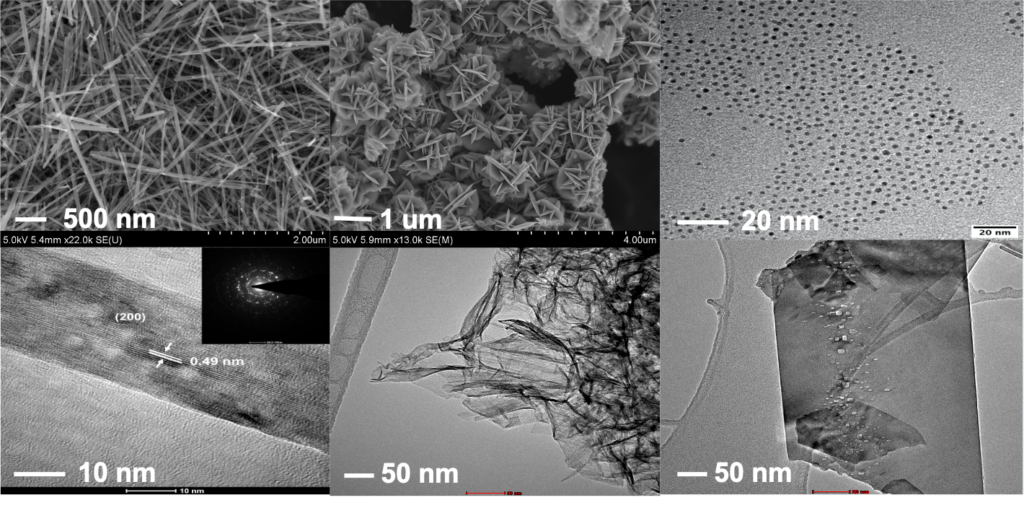
Mechanistic and surface studies
Through a combined analysis of experiment and theory, we investigate reaction models, reaction mechanisms, reaction intermediates, and surface properties using various tools including isotopic tracer experiment, in situ spectroscopies, surface functionalizations, and DFT calculations. We look into reaction mechanisms at an atomistic level so as to achieve finer control of reactivity and selectivity.
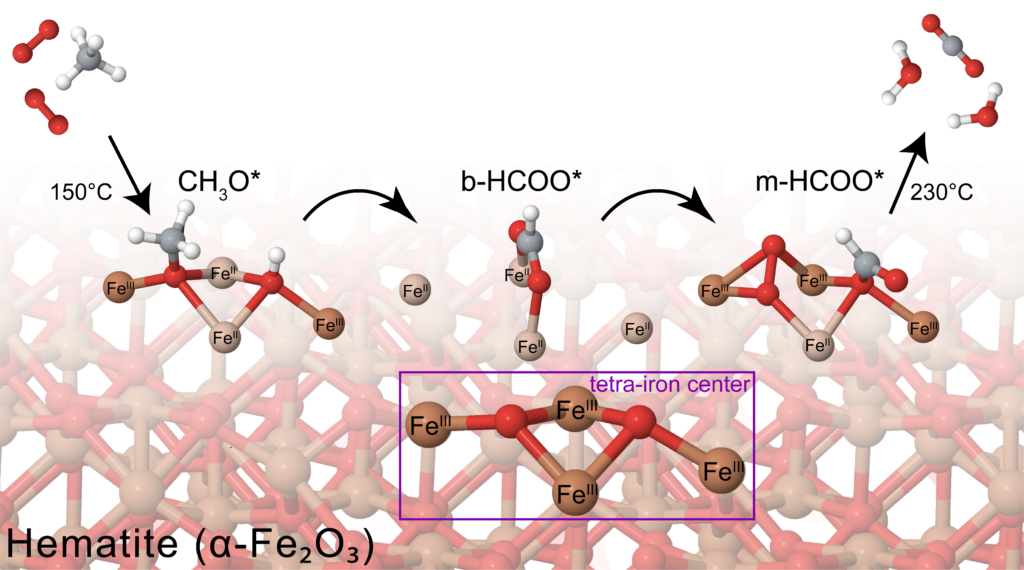
Photothermal catalysis via plasmonic-catalytic hybrid structures
Plasmonic materials exhibit uniquelocalized surface plasmon resonance (LSPR) to convert broadband solar radiation into excited-state charge carriers and perform photochemistry, as well as into thermal energy localized to the reaction sites through plasmon non-radiative relaxation, enabling thermocatalysis. The resulting charge and energy transfer processes would help reduce the activation barrier and boost reaction rates. This emerging technology offers the possibility of directly converting solar energy into chemical energy that can be readily stored and transported without the need for high electrical or thermal energy input, making it particularly suitable for energy-intensive reactions such as CH4 dry reforming (DRM) for reducing both energy and environmental cost as much spent in conventional gas-phase heterogeneous catalysis. Bimetallic plasmonic (Au, Ag, Cu, and Al)-catalytic (Pd, Pt, Ru, and Ir) hybridized structures allow for the incorporation of light into the catalytic processes upon absorption of almost entire solar spectrum. Bimetallic alloys are extremely promising given the tunability of their electronic structures and the plasma optical coupling. Most importantly, the introduction of a catalytic metal, which typically dampening the plasmonic response, could enhance catalytic reactivity and selectivity, as it allows the formation of isolated catalytic sites and/or highly active islands, thus suppressing side reactions and site poisoning.
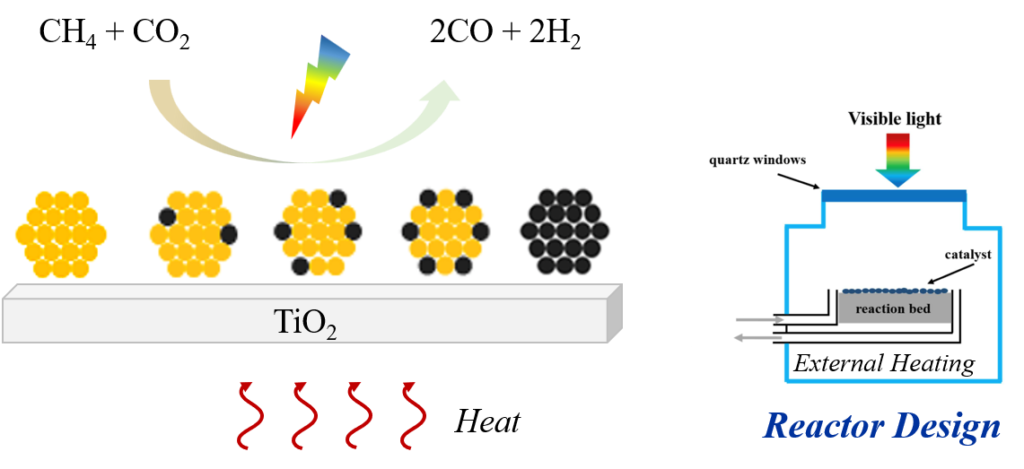
Data-driven Catalyst Design
Recent advances in artificial intelligence have permeated the design process of catalysts via data-driven approaches. In contrast to the traditional QM calculations that rely on chemists’ hard-coded instructions, machine-learning (ML) approaches demand little or no supervision from human operators but learn the latent correlations in the dataset by themselves. Such mechanisms endorse data-driven approaches with a much higher efficiency and lower computational cost than the traditional QM methods. Here, we’re also interested in developing interpretable ML models, in combination with the experiments, to facilitate the catalyst rational design.
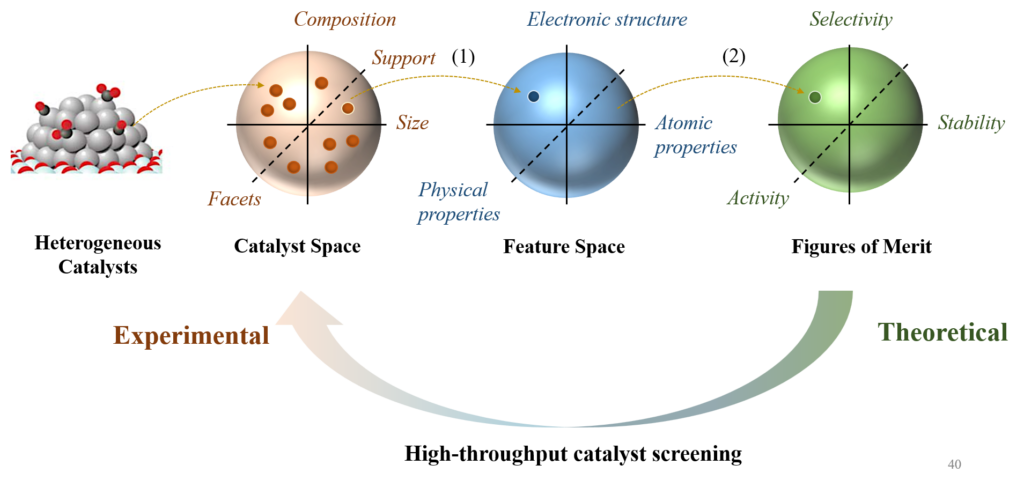